Lepton Flavour
The Standard Model (SM) of Particle Physics – physicist’s best and most tested theory of all the known particles and forces – breaks down at the high energies needed to describe the early universe and is an insufficient theory to adequately describe the universe we observe today. However, studies of lepton flavour (the three flavours of charged leptons being the electron, muon and tau, with each flavour also coming with a neutrino counterpart) are providing corroborating, strengthened evidence of new physics beyond the SM (BSM) that could provide one or more answers. In Manchester, we undertake precision measurements of physical observables of leptons at high-intensity experiments to indirectly search for BSM interactions. Comparing new, precise measurements of the behaviours of electrons, muons and tau leptons with new, precise SM predictions allows for indirect probes of BSM phenomenology by exposing discrepancies between a theoretical prediction and the experiment measurement.
Whereas collider experiments like those at CERN’s Large Hadron Collider (LHC) search for evidence of new physics by looking for the direct production of new particles and interactions (see the ATLAS research on this website), the indirect, high-intensity approach exploits Heisenberg’s uncertainty principle to look for the effects of quantum fluctuations (virtual particles and interactions) that influence the charged leptons being studied. In addition to providing complimentary information to the LHC experiments, such indirect searches for new physics are able to probe energy and mass scales below and orders of magnitude greater than the sensitivity of the LHC, as the collider experiments are limited by the LHC’s beam energy,.
Muons in particular, with their higher mass than the electron and longer lifetime than the tau lepton, are a particularly powerful tool to search for BSM physics. Manchester is actively involved in two muon experiments: the Muon g-2 Experiment and the Mu2e Experiment, both at Fermilab (FNAL) in the USA, and we are also a leading contributor to the theory calculations and SM predictions related to the Muon g-2 Experiment (see the Theory research page on this website). Future plans include electric dipole moment (EDM) searches at the Muon EDM (muEDM) Experiment at the Paul Scherrer Institut (PSI) in Switzerland and proposals for experiments probing a proton EDM.
The Muon g-2 Experiment at Fermilab
Alex Keshavarzi, Mark Lancaster, George Sweetmore
Magnetic moments of charged leptons receive virtual contributions from all particles and interactions, SM or otherwise, and a deviation of the measured value of a magnetic moment from the SM prediction would be a signal of BSM particles or interactions.
The Fermilab Muon g-2 experiment has made the world’s most precise measurement of any quantity at a particle accelerator: the anomalous magnetic moment of the muon (g-2), with a precision of 0.2 ppm. This presently differs from the SM predictions by 2 to 5 standard deviations depending on the methodology used to determine the SM value. Subsequent measurements and refinements in the SM prediction will establish unambiguously whether this is a sign of new physics or not.
The University of Manchester, UCL, and the University of Liverpool were responsible for the Muon g-2 Experiment’s Straw Tracking Detectors. These gaseous straw tracking detectors made detailed measurements of the stored muon beam profile, which are essential for the experiment to achieve its uncertainty goals. Mark Lancaster was co-spokesperson of the Muon g-2 Experiment from 2018-2020.
We also actively contributing to the SM prediction of the Muon g-2, specifically providing the world’s most precise data-driven evaluation of the hadronic vacuum polarisation (HVP). Further details can be found on the Theory research page on this website.
Relevant links
- Fermilab’s Muon g-2 Experiment Website.
- BBC News Article, April 2021: “Muons: ‘Strong’ evidence found for a new force of nature”.
- Run-2 Scientific Publication: “Measurement of the Positive Muon Anomalous Magnetic Moment to 0.20 ppm”.
- Straw Tracking Detector Technical Publication: “The straw tracking detector for the Fermilab Muon g-2 Experiment”.
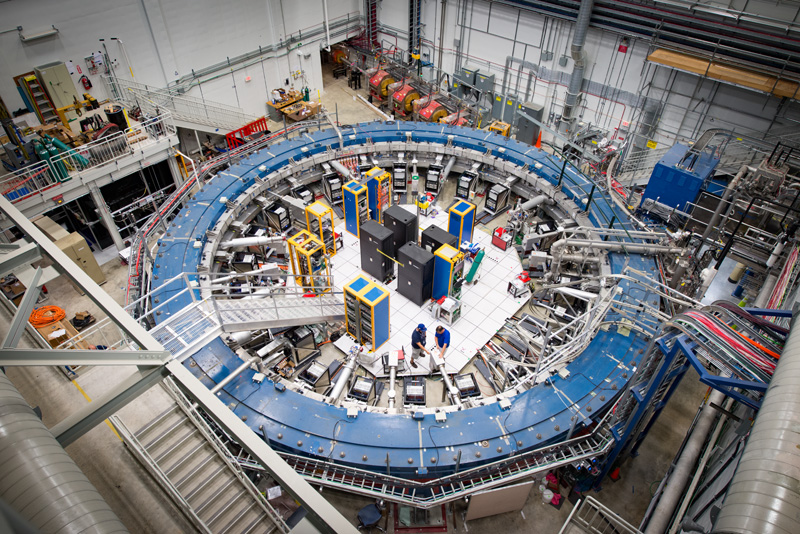
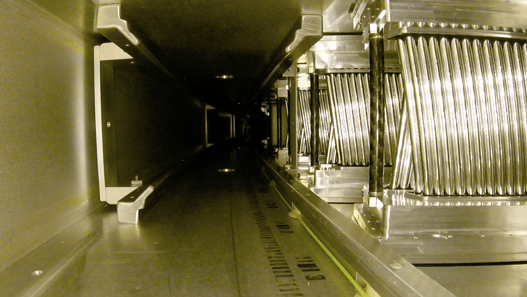
The Mu2e Experiment at Fermilab
Rob Appleby, Silvia Borghi, Alex Keshavarzi, Mark Lancaster, George Sweetmore
Claudia Alvarez-Garcia, Max Jenkinson
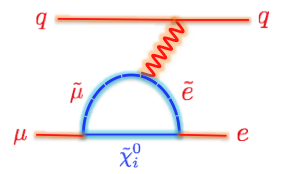
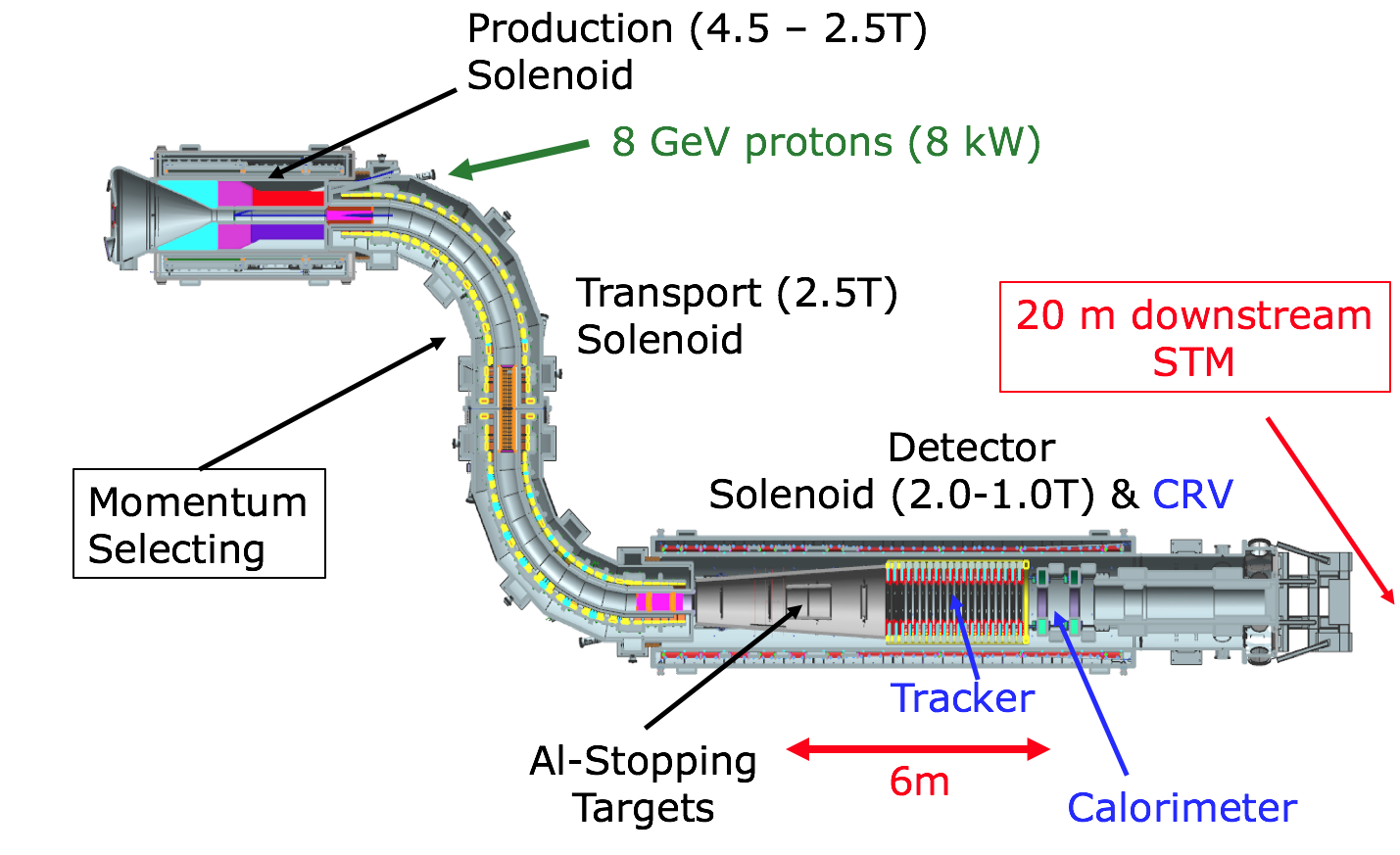
In the SM of particle physics, lepton flavour is always conserved, i.e. there is no interaction that changes the net flavour content of an interaction involving leptons. However, flavour-changing processes of the neutral leptons, neutrinos, have been observed through neutrino oscillations (see the neutrino research page on this website), and so charged lepton flavour violation (CLFV) is also expected. Any observation of CLFV would be unambiguous evidence for BSM physics and next-generation CLFV experiments have a sensitivity to new physics at mass scales far beyond the reach of the LHC, potentially up to 5,000 TeV. They offer a complementary way to search for new physics and to better understand the origin of flavour, which has ramifications for explaining the matter anti-matter asymmetry in the universe.
The Mu2e Experiment Fermilab is seeking to make the first measurement of CLFV by searching for the coherent neutrino-less conversion of a muon to an electron in the field of a nucleus, i.e. μN → eN. Critical to the success of Mu2e is knowledge of the total muon flux, which must be accurately measured to normalise the data and evaluate the performance of the accelerator, solenoids and other detector systems. To this end, the UK is providing the Stopping Target Monitor (STM): a High Purity Germanium (HPGe) detector that measures the X-rays emitted when the muons are captured by the stopping target, which in turn is used to determine the muon flux.
The University of Manchester is responsible for providing the STM’s data acquisition (DAQ) readout software and online monitoring tools. We are also responsible for a collimation system that reduces the radiation damage to the STM (whilst simultaneously ensuring that a sufficient number of X-rays can be recorded), and also the precision alignment of the STM detector and collimator. Future work will include accelerator commissioning and optimisation, commissioning and cross-calibration of the STM and Mu2e’s straw tracking detector, and corresponding analysis of data from both detectors.
Relevant links
Future plans
The muEDM Experiment at PSI
Rob Appleby, Alex Keshavarzi, Mark Lancaster
Searches for BSM sources of CP violation in the charged lepton sector, like a non-zero electric dipole moment (EDM), complement searches in the quark sector (e.g. LHCb, see the LHCb research page on this website) and the neutrino sector (e.g. DUNE, see the neutrino research group page on this website). A direct observation of a non-zero muon EDM would be an unambiguous signal of new physics and could explain the matter-antimatter asymmetry in the universe. The proposed muEDM experiment at the PSI will make use of the frozen-spin technique to improve the current sensitivity to a BSM CP-violating muon EDM by four orders of magnitude.
The University of Manchester, in collaboration with other UK and European institutions, is expected to provide the experiment’s tracking detector and related DAQ. The experiment is expected to begin data-taking in ~ 2027.
Relevant links
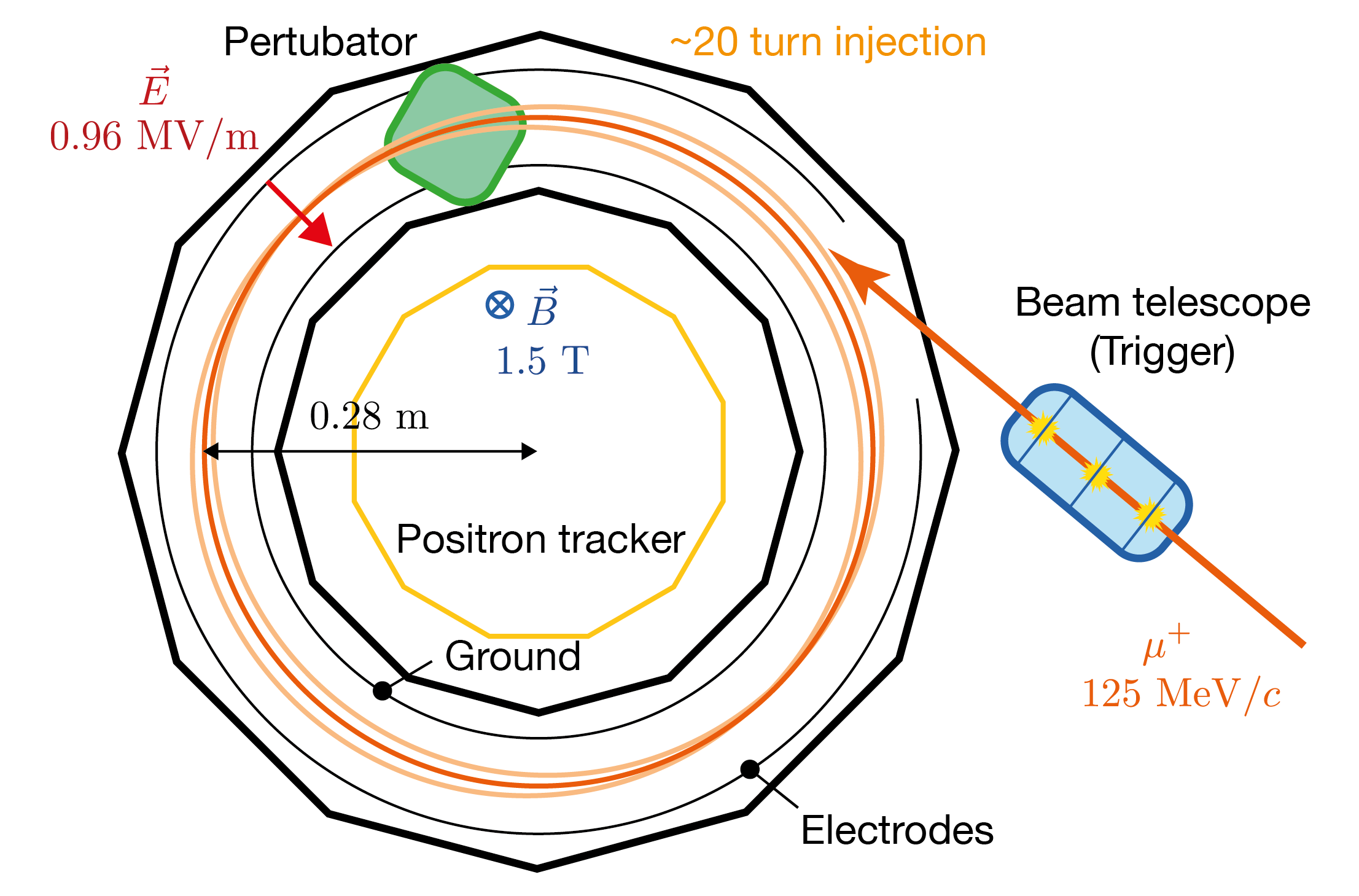
Searching for a proton EDM
Rob Appleby, Alex Keshavarzi, Mark Lancaster
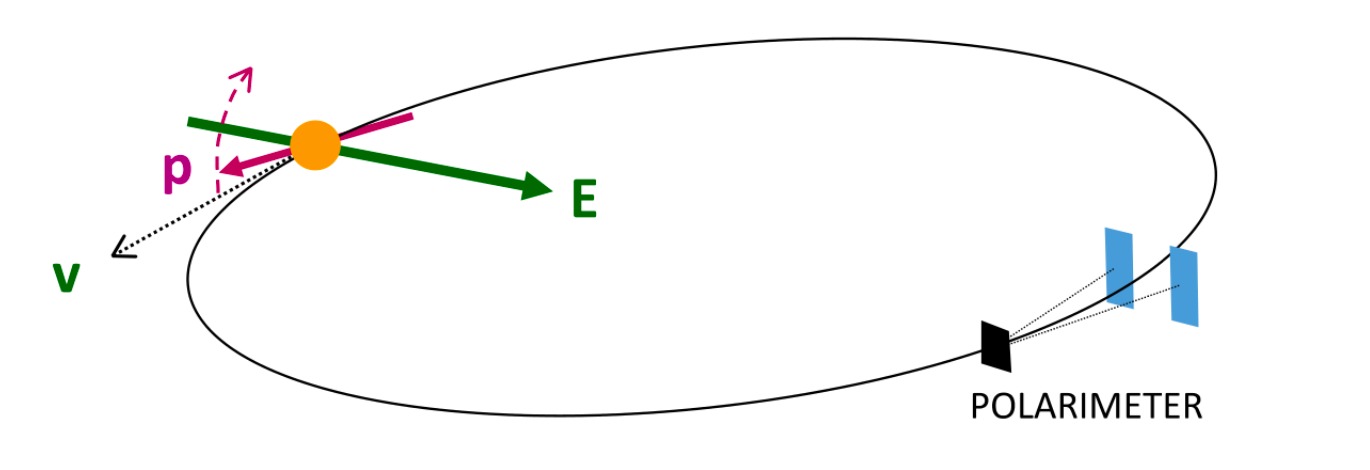
One of the mysteries of particle physics is that the theory of the strong interaction that binds quarks into protons and neutrons cannot explain why the proton and neutron EDM is so small. Consequently, they are yet to be measured. A long postulated explanation is the theoretically predicted existence of a new, light-pseudoscalar particle: the axion, the properties of which also satisfy the requirements of a dark matter candidate (see the Darkside and FASER research group pages on this website). However, the axion has never been experimentally observed.
Observing the axion through a finite proton EDM measurement is of huge potential importance. A proton EDM experiment would be sensitive to a vast array of ranges of dark matter masses and interactions: from very weakly interacting, light particles (30 orders of magnitude below the LHC sensitivity) to strongly interacting, heavy particles (×100 above the mass that the LHC detectors can detect). A sensitivity of 10e−29 e·cm is achievable via the frozen-spin technique in a dedicated proton storage ring.